Research in the Off-Road Diesel Engine Combustion Laboratory is conducted using a single-cylinder, 6.7L research engine. This size class represents one of the most ubiquitous engines in off-road applications. Shown below is the all-metal variant of this engine. We are in the process of developing an optical section in the exhaust runner and a full optical engine variant to enable high-speed imaging; laser-based optical techniques; and detailed study of the thermal, fluid dynamical, and chemical processes occurring inside the combustion chamber.
Diesel combustion research is currently focused in two project areas—science-driven piston bowl designs and catalyst heating operation—discussed below.
Figure 1 Sandia’s single-cylinder 6.7L diesel research engine (shown above) supports the development and calibration of the next generation of off-road diesel combustion systems.
Science-driven piston bowl design
Intelligent design of diesel combustion systems is crucial to promote rapid, efficient combustion with very low pollutant emissions. Combustion system design must account for the shape of the bowl in the piston crown and the injector nozzle parameters, which include the number and geometry of nozzle holes, the injector opening angle, and the location of the nozzle holes relative to the cylinder head.
These parameters influence the interactions between the fuel sprays and the piston bowl walls, establishing the turbulent flow patterns that help mix fuel and air. Improved fuel-air mixing processes lead to faster, cleaner, more efficient combustion. Because the world’s fleet of diesel-powered vehicles is very large, even modest combustion-system design improvements can result in significant reductions in overall CO2 emissions. Scientific study inside state-of-the-art diesel combustion chambers produces fundamental insights necessary to improve piston bowl shapes.
The project focuses on a specific type of commonly used diesel-piston design with stepped-lip or chamfered-lip bowls (shown above). High-speed imaging and thermodynamic experiments in an optical diesel engine have provided evidence that spray-wall interactions can create strong, long-lived vortices under some conditions. The appearance of these vortices correlates with faster, more efficient combustion and reductions in soot emissions (as illustrated below). However, the vortices become weaker as the fuel injection timing is advanced toward top-dead center (TDC), which is necessary to achieve peak efficiency.
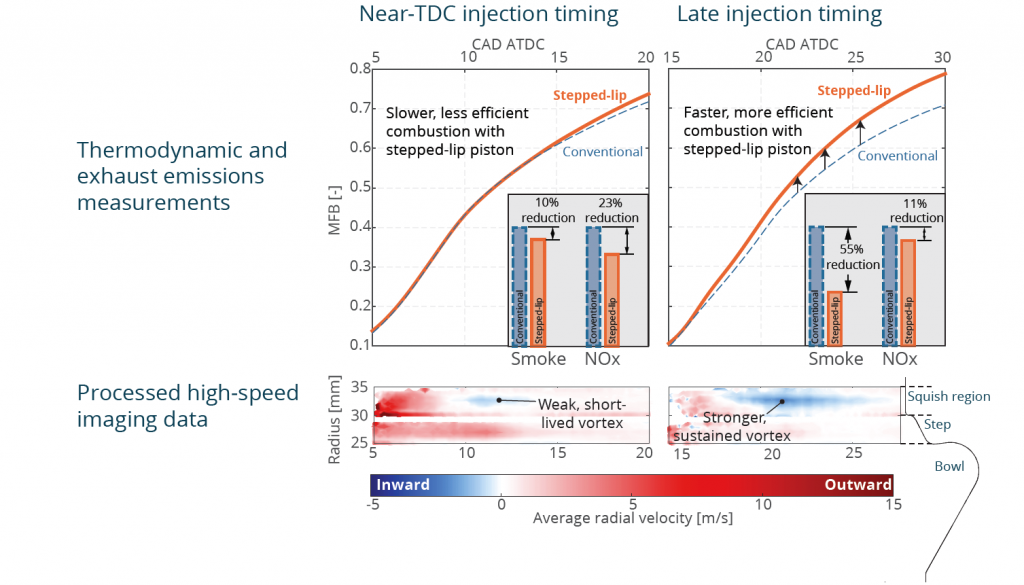
Researchers have modeled this complex turbulent flow behavior using numerical simulations. In-depth analysis of the results has provided insight into the physics responsible for the observed vortex behavior, leading to the following hypothesis: If a change in combustion system design creates stronger, longer-lived vortices at near-TDC injection timings, then peak efficiency will be improved while soot emissions are reduced. A modified stepped-lip piston design has been conceived to test this hypothesis, with the resulting design called a dimpled stepped-lip (DSL) piston.
Researchers’ simulations predict that DSL pistons promote vortex strength and longevity for injection timings near TDC. Using simulation tools, we are developing a DSL piston design for Sandia’s off-road diesel research engine and performing experiments with a metal version of the DSL piston to quantify its benefits on efficiency and emissions over a wide range of operating conditions.
Catalyst Heating Operation
Meeting current and future exhaust emissions regulations requires the use of after-treatment systems in diesel engine exhaust. These systems use catalysts to help convert harmful criteria pollutants into CO2 and water, but the catalysts are only effective once they have reached their so-called “light-off” temperature. A cold-started engine must be operated to heat up the exhaust catalysts to their light-off temperatures as quickly and efficiently as possible, while minimizing pollutant emissions that will leave the exhaust system untreated. When operated at low loads, the engine exhaust temperatures must remain high enough to keep the catalysts above their light-off temperature. Delivering clean, compliant, efficient diesel engines to market requires effective catalyst heating and thermal management strategies. At the CRF, we are improving our understanding of how in-cylinder processes during catalyst heating operation work to create tradeoffs between exhaust heat, pollutant emissions, and efficiency. Further investigations help CRF researchers understand how changes in fuel properties influence these tradeoffs.