The laser diagnostic and mass spectrometry expertise at the CRF is also being applied to probe the chemical structure of nonequilibrium reacting plasmas. In particular, the influence of excited molecular and atomic species formed in a plasma discharge on fuel oxidation, plasma stability, and the reforming of CO2 to produce syngas are being investigated. This work is part of a broader Sandia effort as part of the DOE Fusion Energy Science-funded Plasma Research Facility.
Selected Research
Scientific Achievement
Scientists from Sandia National Laboratories have designed and demonstrated a new apparatus for the formation of ultracold laser-induced plasmas and diagnostics to investigate plasma evolution on nanosecond time scales. They are able to monitor chemistry within the plasma as well as the electron velocity distribution by extracting and imaging cations, anions, or electrons from the plasma using sub-nanosecond electrical pulses. Analysis of the images enables one to determine the intensity and temperature of the plasma as a function of time.
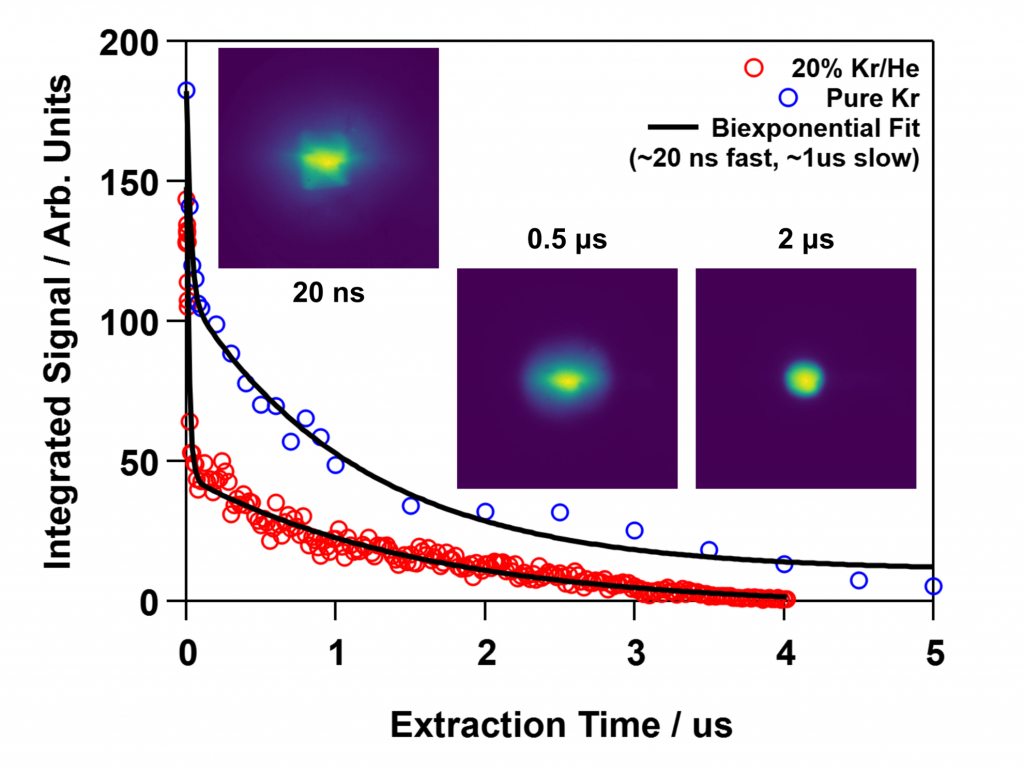
Significance and Impact
- This work demonstrates a new technique for measurements of the electron temperature within a plasma
- This technique enables investigations of the temporal evolution of plasma characteristics with nanosecond resolution.
- There are many situations that require quantification of the chemistry and dynamics of a plasma, such as understanding properties of plasmas used in chemical vapor deposition.
Research Details
- An atomic beam of cold Kr atoms was formed in a pulsed supersonic expansion, and a 214-nm laser pulse resonantly ionized the Kr within a field free region. The resulting Kr cations trapped the photoelectrons to form a transient plasma.
- Electrons from the plasma were imaged onto a position sensitive microchannel plate detector by applying an 800 picosecond, 6000 volt pulse that extracted the electrons and drove them through an electrostatic lens.
- The velocity mapped images allow one to obtain the electron velocity distribution in the plasma. By fitting this distribution to a temperature, one can observe temperature variations with nanosecond resolution.
Publication
Smoll, E. J.; Jana, I.; Frank, J. H.; Chandler, D. W. Velocity-mapped imaging of electron dynamics in an ultracold laser-induced plasma. Physical Review A 2023, 108 (4), L041301. DOI: https://doi.org/10.1103/PhysRevA.108.L041301.
Researchers demonstrate quantitative imaging of the methyl radical (CH3) in a nanosecond pulsed plasma using photofragmentation laser-induced fluorescence.
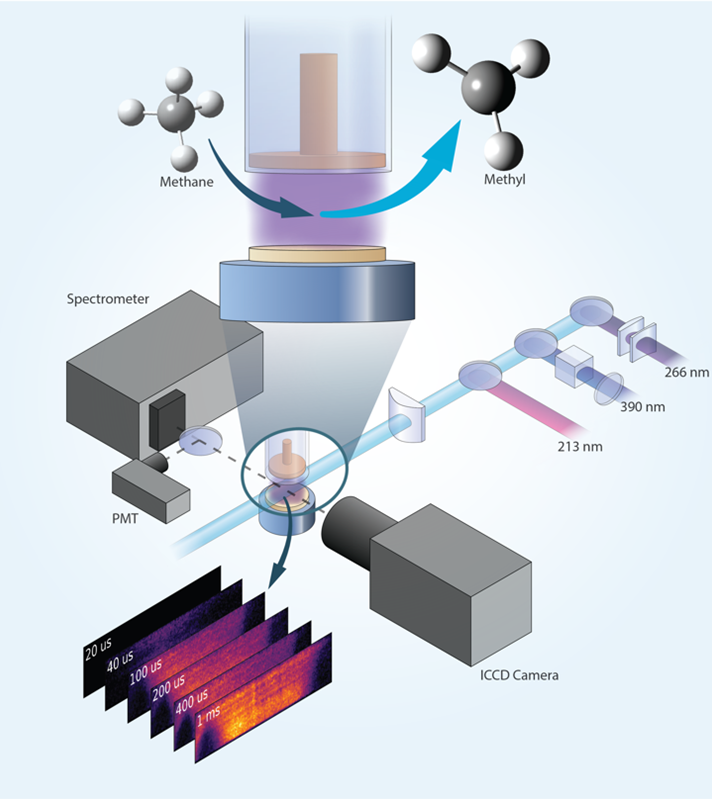
The Science
Low-temperature plasmas can facilitate chemical conversion of hydrocarbons while avoiding use of high-temperature reactors. Methyl (CH3) is a key reactive molecule in plasma hydrocarbon chemistry. For example, it is formed by removal of a hydrogen atom from methane (CH4). Scientists need imaging measurements of methyl distributions to better understand chemical reactions of hydrocarbons in plasmas. Laser diagnostics provide non-intrusive measurements in plasmas. However, laser imaging of methyl using a conventional approach of laser-induced fluorescence is problematic because methyl falls apart after laser excitation. Scientists have overcome this limitation by detecting the CH fragment that is formed when methyl falls apart.
The Impact
The demonstration of 2D imaging measurements of the methyl radical in a low-temperature plasma opens new opportunities to understand the formation and destruction of a key molecule in hydrocarbon chemistry of plasmas. Studies using this diagnostic technique could provide new insights into how plasmas promote chemical reactions in important applications, such as plasma-assisted combustion, catalysis, and reforming. A deeper understanding and control of these processes is needed to develop new approaches to plasma-assisted energy conversion and chemical synthesis, such as the generation of higher value hydrocarbons from inexpensive abundant hydrocarbon fuels.
Summary
The methyl radical plays a central role in plasma-assisted hydrocarbon chemistry but is challenging to detect due to its high reactivity and strongly pre-dissociative electronically excited states. Researchers at a DOE/FES Low Temperature Plasma Research Facility have demonstrated quantitative 2D imaging of methyl profiles in a plasma using photo-fragmentation laser-induced fluorescence (PF-LIF). This technique provides temporally and spatially resolved measurements of local methyl distributions, including in near-surface regions that are important for plasma-surface interactions such as plasma-assisted catalysis. The technique relies on laser photo-dissociation of methyl to produce CH fragments. These photofragments are then detected with LIF imaging using a second laser to excite CH at 390nm, and fluorescence from CH is detected near 430nm. This non-resonant detection scheme enables interrogation close to a surface. The PF-LIF diagnostic is calibrated by producing a known amount of methyl through photo-dissociation of acetone vapor in a calibration gas mixture using a third laser. PF-LIF imaging of methyl production is demonstrated in methane-containing nanosecond pulsed plasmas with calibrated measurements obtained in a diffuse, plane-to-plane discharge. Relative methyl measurements in a filamentary plane-to-plane discharge and a plasma jet reveal highly localized intense production of methyl. The utility of the PF-LIF technique is further demonstrated by combining methyl measurements with formaldehyde LIF imaging to capture correlations between methyl and formaldehyde.
Publication
van den Bekerom, D., Richards, C., Huang, E., Adamovich, I. & Frank, J. H. 2D imaging of absolute methyl concentrations in nanosecond pulsed plasma by photo-fragmentation laser-induced fluorescence. Plasma Sources Sci. Technol. 31, 095018 (2022). [https://doi.org:10.1088/1361-6595/ac8f6c]
Researchers demonstrate quantitative imaging of hydrogen peroxide (H2O2) in a nanosecond pulsed humid plasma using photofragmentation laser-induced fluorescence.
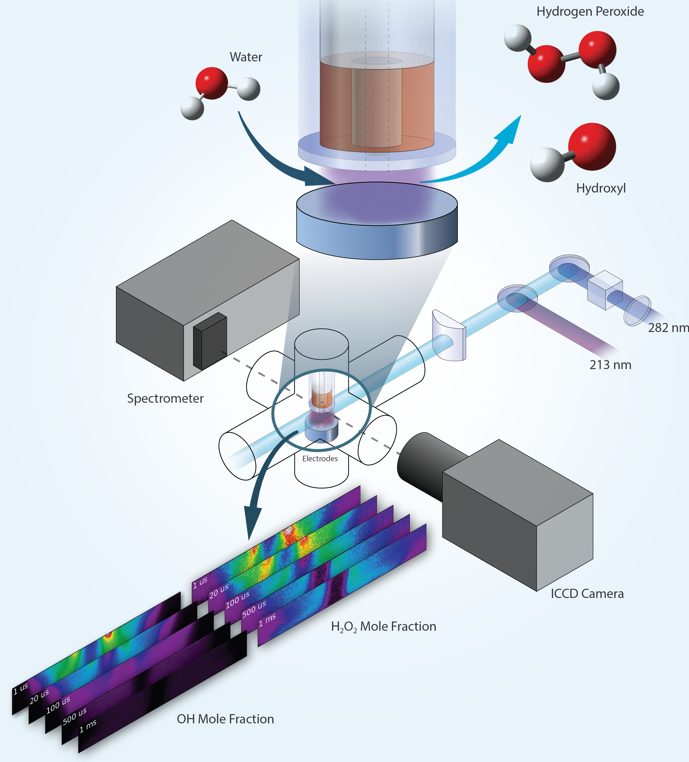
The Science
Low-temperature plasmas can facilitate chemical reactions without the need for high-temperature reactors that are precluded in temperature sensitive applications, such as biomedical treatments. Hydrogen peroxide (H2O2) and the hydroxyl radical (OH) are key reactive molecules in plasma water chemistry and can promote decontamination, wound healing, and other biomedical treatments. Scientists need imaging measurements of the distributions of these molecules to better understand chemical reactions in water-containing plasmas and the interactions of plasmas with aqueous solutions. Laser diagnostics provide non-intrusive measurements in plasmas. However, laser imaging of hydrogen peroxide using a conventional approach of laser-induced fluorescence is problematic because hydrogen peroxide falls apart after laser excitation. Scientists have overcome this limitation by detecting the OH fragments that are formed when hydrogen peroxide falls apart.
The Impact
The demonstration of 2D imaging measurements of hydrogen peroxide and the hydroxyl radical in a low-temperature plasma opens new opportunities to understand the formation, consumption, and transport of key molecules in water chemistry of plasmas. Studies using this diagnostic technique could provide new insights into how plasmas promote chemical reactions in important applications, such as plasma-assisted sterilization, decontamination, and biomedical treatments. A deeper understanding of these processes is needed to advance plasma applications and enable control of plasma sources to tailor the delivery of key species.
Summary
Hydrogen peroxide and the hydroxyl radical play central roles in plasma water chemistry, but hydrogen peroxide is challenging to detect due to its high reactivity and strongly pre-dissociative electronically excited states. Researchers at a DOE/FES Low Temperature Plasma Research Facility have demonstrated quantitative 2D imaging of hydrogen peroxide profiles in a humid plasma using photo-fragmentation laser-induced fluorescence (PF-LIF). This technique provides temporally and spatially resolved measurements of local hydrogen peroxide distributions, including in near-surface regions that are important for plasma-liquid or plasma-surface interactions such as treatment of tissue or sterilization/decontamination. The technique relies on laser photo-dissociation of hydrogen peroxide at 213nm to produce OH fragments. These photofragments are then detected with LIF imaging using a second laser to excite OH at 282nm, and fluorescence from OH is detected near 310nm. This non-resonant detection scheme enables interrogation close to a surface. The PF-LIF diagnostic is calibrated using a reference gas mixture containing a known amount of hydrogen peroxide. PF-LIF imaging is used to measure 2D profiles of hydrogen peroxide mole fraction in a humid nanosecond pulsed dielectric barrier discharge. The utility of the PF-LIF technique is further demonstrated by combining hydrogen peroxide measurements with OH-LIF imaging to capture correlations between hydrogen peroxide and hydroxyl radical mole fractions.
Publication
van den Bekerom, D., Tahiyat, M. M., Huang, E., Frank, J. H., Farouk, T. I., 2D-imaging of absolute OH and H2O2 profiles in a He–H2O nanosecond pulsed dielectric barrier discharge by photo-fragmentation laser-induced fluorescence. Plasma Sources Sci. Technol. 32, 015006 (2023). [https://doi.org:10.1088/1361-6595/acaa53]